Introduction
Waste-to-Energy (WtE) projects represent a critical intersection of waste management and renewable energy production. By transforming the organic and inorganic waste materials that would otherwise populate landfills into various forms of energy, these projects offer a pragmatic solution to the dual challenges of waste surplus and energy scarcity. WtE technologies such as combustion, gasification, pyrolysis, anaerobic digestion, and landfill gas recovery convert waste into electricity, heat, or fuel with varying degrees of efficiency and environmental impact.
Amid growing global concern over climate change, WtE projects also play a pivotal role in carbon management through the generation of carbon credits. These credits are tradable certificates that represent the prevention or removal of one tonne of carbon dioxide from the atmosphere, serving as a currency in the carbon market. As nations and corporations alike strive to reduce their carbon footprints, understanding the interaction between WtE projects and carbon credit generation is essential for fostering sustainable practices.
Understanding Carbon Credits
Carbon credits are at the heart of market-based strategies to mitigate greenhouse gases. They operate under the principle that companies or projects that reduce or sequester carbon emissions can earn credits that can be sold to other entities unable to reduce their emissions directly. These transactions are facilitated by carbon markets, which can be either regulated under government mandates, such as cap-and-trade programs, or voluntary, where companies participate to meet self-imposed carbon reduction goals or improve their public image.
In regulated markets, governments set a cap on the total amount of greenhouse gases that can be emitted. Companies are then given or can buy a certain number of credits. If they exceed their emissions quota, they must purchase additional credits; if they emit less, they can sell their surplus credits. In voluntary markets, carbon credits are often used by companies to offset emissions, such as those from corporate travel, by investing in projects that reduce carbon emissions elsewhere. Learn more about this topic here.
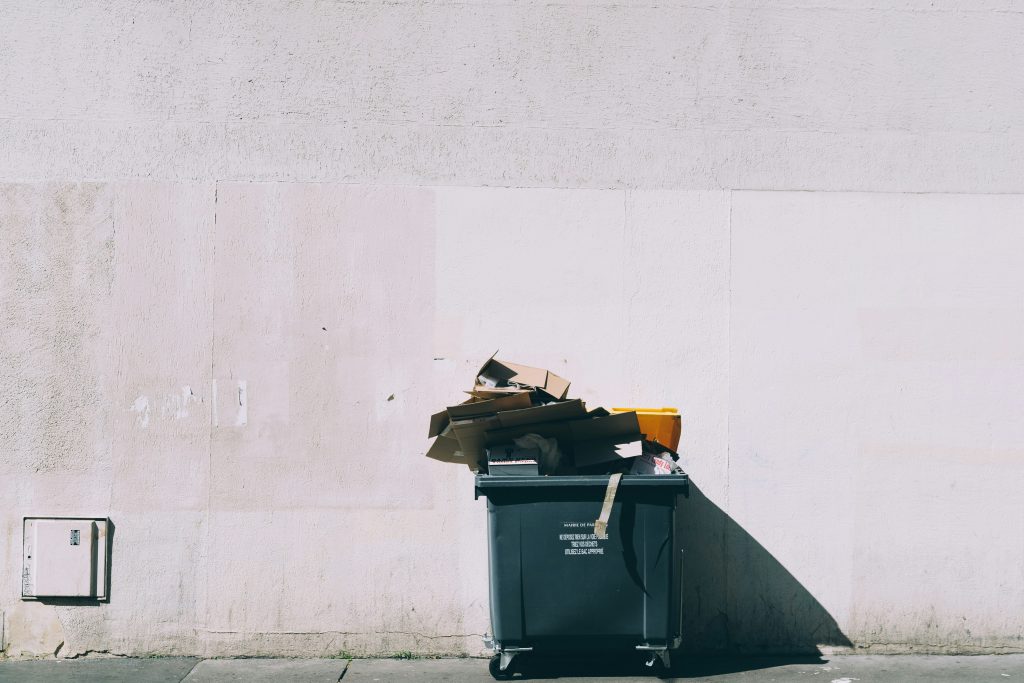
Waste-to-Energy Technologies and Their Carbon Impact
Waste-to-Energy (WtE) technologies are integral in the management of municipal solid waste, turning what was once merely discarded material into valuable energy resources. These technologies not only help in reducing the volume of waste but also contribute significantly to the reduction of greenhouse gas emissions through various mechanisms. Let’s delve into the specifics of these technologies and their impacts on carbon emissions:
- Combustion: This is the most widely used WtE technology, involving the incineration of organic waste materials to produce electricity and heat. The process burns waste at high temperatures, and the heat generated boils water, creating steam that drives turbines to produce electricity. Despite its efficiency in reducing waste volume by up to 90%, combustion can emit pollutants like nitrogen oxides, sulfur dioxide, and particulate matter if not properly controlled. Modern combustion facilities, however, are equipped with advanced emission control systems that significantly reduce these pollutants. Moreover, by diverting waste from landfills, combustion reduces methane emissions, a potent greenhouse gas that is released during the anaerobic decomposition of organic waste in landfills.
- Gasification: This technology involves subjecting waste to high temperatures in a low-oxygen environment, converting organic materials into a synthesis gas (syngas), which is primarily composed of hydrogen and carbon monoxide. Syngas can be burned directly to generate electricity or can be processed into other forms of fuel. Gasification is particularly noted for its ability to handle a variety of wastes, including biomass, plastics, and even hazardous wastes. It produces fewer and cleaner emissions compared to combustion and has the potential to capture carbon from the syngas before it is utilized, enhancing its greenhouse gas mitigation potential.
- Pyrolysis: Similar to gasification, pyrolysis processes waste materials at high temperatures in the absence of oxygen. Instead of producing syngas, pyrolysis yields a solid residue (char), liquid (tar), and gas, which can be refined into fuels or chemical feedstocks. The char can sequester carbon in soil, contributing further to carbon reduction. Pyrolysis is particularly promising for processing plastic wastes, transforming them into commercial hydrocarbon products.
- Anaerobic Digestion: This biological process breaks down organic waste in the absence of oxygen, producing biogas (mainly methane and carbon dioxide) and digestate, which can be used as a fertilizer. The biogas produced is a renewable energy source that can replace natural gas for heating or electricity generation. Anaerobic digestion is highly effective in reducing greenhouse gas emissions by capturing methane that would otherwise escape into the atmosphere from decaying waste.
- Landfill Gas Capture: While not a direct conversion of waste to energy, landfill gas capture systems collect the methane produced naturally as organic waste decomposes in landfills. The captured methane can be used to generate electricity or heat or can be processed into renewable natural gas. By capturing and utilizing this methane, landfill gas systems prevent a significant source of greenhouse gas emissions and utilize it as a clean energy source.
Each of these technologies contributes differently to the reduction of carbon emissions. By diverting waste from landfills, they reduce methane emissions. By replacing fossil fuels with the energy they produce, they decrease carbon dioxide emissions. In addition to mitigating climate change, these technologies also help in managing waste more sustainably, marking a significant shift in how societies value and utilize what was once considered mere trash.
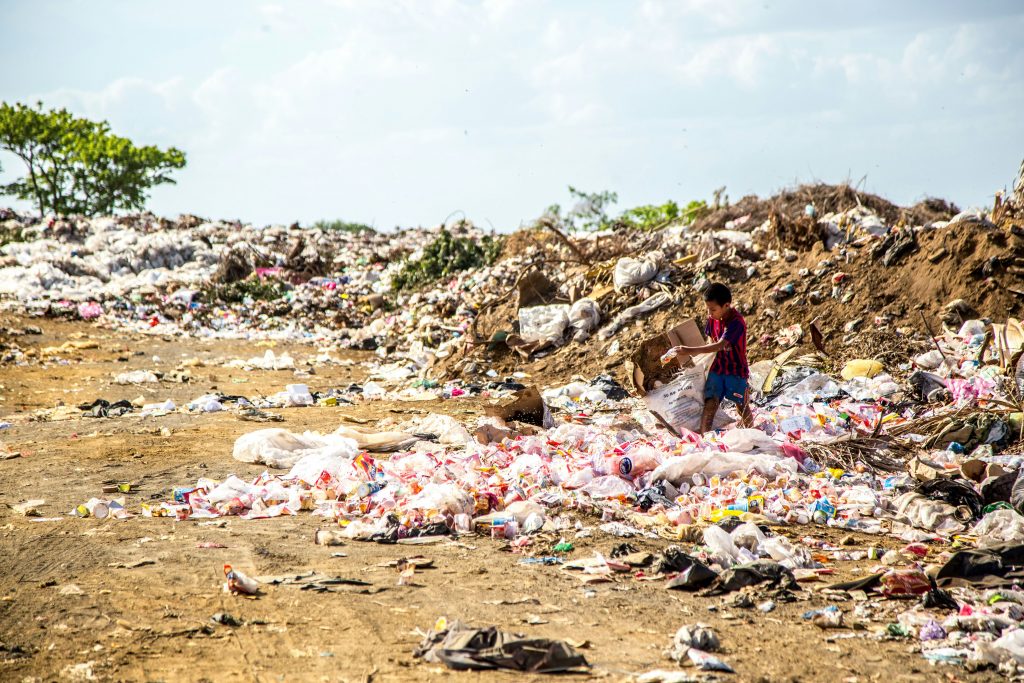
Generating Carbon Credits Through WtE Projects
Waste-to-Energy (WtE) projects offer a unique opportunity to combat climate change by converting waste into energy while simultaneously generating carbon credits. These credits can play a crucial role in the global carbon market, providing financial incentives for reducing greenhouse gas emissions. Here, we’ll explore the mechanisms through which WtE projects generate carbon credits, the verification processes involved, and examples of successful implementations.
Mechanisms for Carbon Credit Generation
WtE projects generate carbon credits by demonstrating tangible reductions in greenhouse gas emissions. This is achieved through several key mechanisms:
- Methane Reduction: WtE technologies like anaerobic digestion and landfill gas capture prevent methane, a potent greenhouse gas, from being released into the atmosphere. By capturing methane that would otherwise contribute to global warming if released from landfills, these projects can claim carbon credits for every tonne of CO2 equivalent they prevent.
- Displacement of Fossil Fuels: WtE projects often produce electricity or heat that can replace those derived from fossil fuels. By using waste as a fuel source, these projects reduce the overall demand for fossil fuels, thereby reducing associated CO2 emissions. Carbon credits are generated based on the amount of fossil fuel energy displaced by WtE-generated energy.
- Energy Recovery: By converting waste materials into energy, WtE processes decrease the need for additional energy production and the consequent greenhouse gas emissions. This energy recovery is particularly significant in areas where electricity production relies heavily on coal or other high-emission sources.
Verification and Certification of Carbon Credits
To ensure that carbon credits represent genuine emission reductions, WtE projects must undergo rigorous verification processes:
- Baseline Establishment: A baseline of emissions must be established, which estimates the amount of greenhouse gas that would have been emitted had the WtE project not been implemented.
- Monitoring and Reporting: Continuous monitoring is required to assess the actual emissions from the WtE project. This data is then compared against the baseline to calculate the net reductions in emissions. Detailed reports are prepared and submitted to independent verifying bodies.
- Third-Party Verification: Independent third-party verifiers assess the project’s compliance with established methodologies and the accuracy of its emission reduction reports. Only after a successful verification can the project issue carbon credits.
- Certification Standards: Credits are certified under standards such as the Verified Carbon Standard, the Gold Standard, or those established by local governments under regulatory frameworks like the Clean Development Mechanism (CDM) of the Kyoto Protocol or the mechanisms developed under the Paris Agreement.
Examples of Successful Carbon Credits Generation
One such example is the partnership between CoreZero, a Miami-based climate tech company, and The Mexican Foodbanking Network. This partnership has developed a method to create carbon credits by preventing 221,800 tons of carbon emissions from food waste through the VCM. This project represents the world’s first carbon credits from food rescue and provides a new option for offsetting carbon emissions.
Another example is the use of anaerobic digesters to convert organic waste into biogas, which can be used as a renewable energy source. This process not only reduces the amount of waste sent to landfills but also generates carbon credits that can be traded or sold to offset carbon emissions.
Bio-CNG projects are also eligible for carbon credits under the Carbon Credit Trading Scheme 2023 in India. The carbon credits earned from producing a tonne of Bio-CNG vary between 16 and 25, depending on the type of feedstock used. Each credit holds a value between $5 and $15 in the current international market. For a 5-tonne-per-day Bio-CNG plant, this translates to an annual revenue ranging from Rs 14,94,935 to Rs 4,48,41,071. The potential of this market is enormous, with developing countries leading the way.
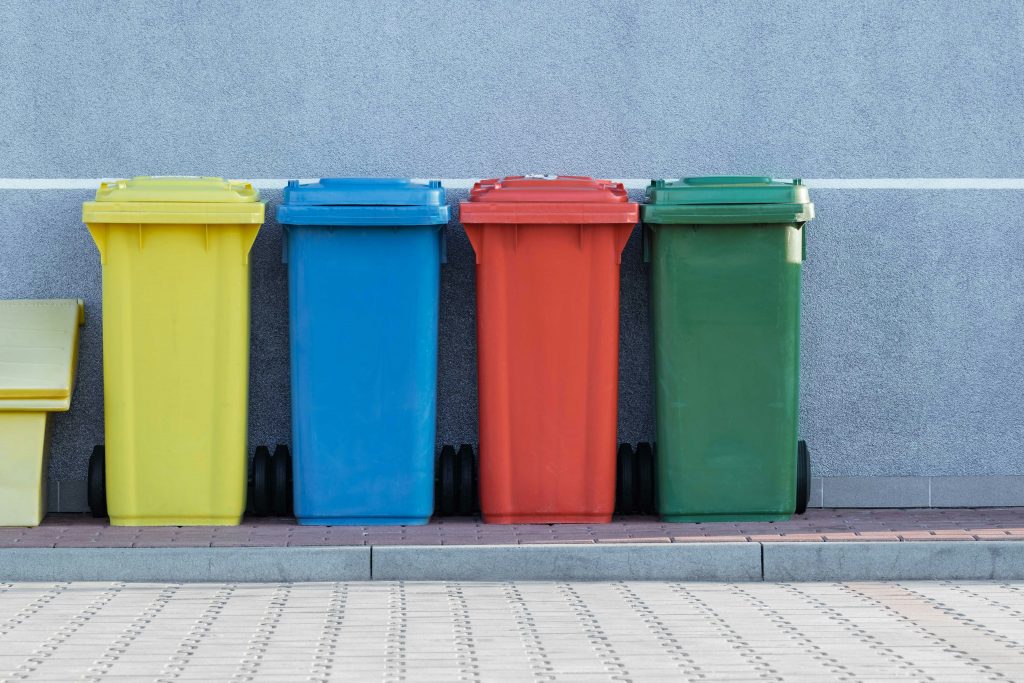
Future Prospects of WtE Projects and Carbon Trading
As global concerns about climate change and waste management continue to intensify, the role of Waste-to-Energy (WtE) projects is poised to become even more significant. The integration of these projects into carbon trading schemes also presents a promising avenue for promoting environmental sustainability and economic growth. Here, we’ll explore the future trends, potential technological advancements, policy developments, and market dynamics that are likely to influence the trajectory of WtE projects and carbon trading.
Technological Advancements
Innovation in WtE technology is crucial for increasing the efficiency and environmental friendliness of these projects. Future advancements are expected in several key areas:
- Efficiency Improvements: Ongoing research aims to enhance the energy conversion rates of WtE facilities, reducing the amount of waste required to produce the same amount of energy. This would directly decrease operational costs and increase competitiveness with traditional energy sources.
- Emissions Reduction: Advanced emission control technologies are being developed to minimize the release of pollutants, including particulates, dioxins, and heavy metals. These improvements are critical for meeting stringent environmental regulations and making WtE a more attractive option for generating carbon credits.
- Gasification and Pyrolysis: These technologies are expected to gain traction due to their ability to process diverse waste streams and produce cleaner energy. Innovations could make these processes more viable at smaller scales or for specific types of waste, broadening their application.
- Carbon Capture and Storage (CCS): Integrating CCS technology into WtE plants could dramatically reduce their net carbon emissions, potentially turning them into negative emissions facilities. This would enhance their value in carbon markets and support global carbon reduction goals.
Policy and Regulatory Developments
The future of WtE projects is also heavily influenced by policy and regulatory frameworks, which can either foster or hinder their growth:
- Incentives and Subsidies: Governments may introduce more incentives for WtE projects, such as tax breaks, subsidies, and grants, especially in regions facing significant waste management challenges.
- Carbon Pricing: As more countries adopt and strengthen carbon pricing mechanisms, the economic benefits of WtE projects in terms of carbon credit generation could become more pronounced. This would encourage investment in WtE technologies and operations.
- International Agreements: Global climate agreements, following on the Paris Agreement, could set more explicit targets for waste management and renewable energy, directly benefiting WtE projects.
- Standardization of Carbon Credits: Efforts to standardize and regulate the carbon market could increase transparency and trust in carbon trading, enhancing the credibility of carbon credits generated from WtE projects.
Market Dynamics
The carbon trading market is dynamic and influenced by a variety of factors that can affect the valuation and demand for carbon credits from WtE projects:
- Voluntary Carbon Markets: As corporate responsibility and sustainability goals become more ambitious, companies might increase their participation in voluntary carbon markets, driving up demand for credits from WtE projects.
- Integration with Recycling and Circular Economy: There is growing emphasis on integrating WtE with recycling efforts and broader circular economy principles. This integration could help balance waste reduction with energy recovery, aligning WtE projects more closely with sustainability objectives.
- Public Perception and Acceptance: The success of WtE projects also hinges on public perception. Increased awareness of the benefits of WtE in terms of both waste reduction and clean energy production can lead to greater community support and acceptance.
Conclusion
Waste-to-Energy (WtE) projects have emerged as pivotal solutions in the global push towards sustainability, adeptly addressing the dual challenges of managing escalating waste volumes and reducing reliance on fossil fuels. By transforming waste into energy, these projects contribute significantly to environmental conservation, resource efficiency, and energy security. Moreover, their ability to generate carbon credits adds a valuable dimension, aligning economic incentives with climate action goals.
As the world grapples with the pressing imperatives of climate change and waste management, the integration of WtE technologies in carbon trading markets is not just beneficial but essential. These projects offer a pragmatic approach to curbing greenhouse gas emissions by harnessing the untapped energy potential of waste, thus turning a perennial problem into a powerful resource. This conversion process not only helps reduce landfill use and methane emissions but also provides a renewable energy source that can displace fossil fuels, thereby mitigating carbon dioxide emissions.
Looking forward, the prospects for WtE projects are robust and filled with opportunities for innovation and growth. Technological advancements are expected to enhance the efficiency and environmental sustainability of these projects, making them more appealing and effective. Policy developments that provide clearer incentives, regulatory support, and robust carbon pricing mechanisms will further bolster the economic viability of WtE initiatives. Additionally, as carbon markets mature and expand, the demand for verified carbon credits from reliable and impactful projects like WtE will likely increase.
References
- BP (n.d.) ‘Our carbon offset project portfolio’. Available at: https://www.bp.com/en_gb/target-neutral/home/our-carbon-offset-project-portfolio/offset-projects-from-our-previous-portfolios.html
- Carbon Credits (n.d.) ‘Turning 1.3 billion tons of food waste into carbon credits’. Available at: https://carboncredits.com/turning-1-3-billion-tons-of-food-waste-into-carbon-credits/
- Cleanhub (n.d.) ‘Carbon credits vs. plastic credits’. Available at: https://blog.cleanhub.com/carbon-credits-vs-plastic-credits
- Climate Impact (n.d.) ‘Carbon credits explained: What they are and how they work’. Available at: https://www.climateimpact.com/services-projects/carbon-credits-explained-what-they-are-and-how-they-work/
- Down To Earth (n.d.) ‘Bio CNG projects as carbon credit generators’. Available at: https://www.downtoearth.org.in/blog/energy/bio-cng-projects-as-carbon-credit-generators-90386
- Drawdown (n.d.) ‘Waste-to-energy’. Available at: https://drawdown.org/solutions/waste-to-energy
- EFW Network (n.d.) ‘The time for carbon capture in energy from waste is now’. Available at: https://network.efwconference.com/posts/the-time-for-carbon-capture-in-energy-from-waste-is-now-d78a9dcd-b001-4f99-ac52-9f39942cbe5a
- EIA (n.d.) ‘Waste-to-energy in-depth’. Available at: https://www.eia.gov/energyexplained/biomass/waste-to-energy-in-depth.php
- EIC (n.d.) ‘4 types of carbon offset projects’. Available at: https://eic.co.uk/4-types-of-carbon-offset-projects/
- EPA (n.d.) ‘Energy recovery from combustion of municipal solid waste (MSW)’. Available at: https://www.epa.gov/smm/energy-recovery-combustion-municipal-solid-waste-msw
- GNPW (n.d.) ‘How do landfills generate carbon credit?’. Available at: https://www.gnpw.com.br/en/energy/how-do-landfills-generate-carbon-credit/
- LinkedIn (n.d.) ‘The significance of carbon credits for renewable energy companies’. Available at: https://www.linkedin.com/pulse/significance-carbon-credits-renewable-energy-companies
- PreScouter (2017) ‘Waste-to-energy technologies available’. Available at: https://www.prescouter.com/2017/10/waste-to-energy-technologies-available/
- SafetyCulture (n.d.) ‘Waste management system’. Available at: https://safetyculture.com/topics/waste-management-system/
- S&P Global (n.d.) ‘Voluntary carbon markets: Pricing, participants, trading CORSIA credits’. Available at: https://www.spglobal.com/commodityinsights/en/market-insights/blogs/energy-transition/061021-voluntary-carbon-markets-pricing-participants-trading-corsia-credits
- ScienceDirect (n.d.) ‘No title’. Available at: https://www.sciencedirect.com/science/article/pii/S0956053X23006955
- Valuer (n.d.) ‘Top innovative technologies in waste-to-energy’. Available at: https://www.valuer.ai/blog/top-innovative-technologies-in-waste-to-energy
- Wikipedia (n.d.) ‘Incineration’. Available at: https://en.wikipedia.org/wiki/Incineration
- Wikipedia (n.d.) ‘Waste-to-energy’. Available at: https://en.wikipedia.org/wiki/Waste-to-energy
- Wikipedia (n.d.) ‘Waste-to-energy plant’. Available at: https://en.wikipedia.org/wiki/Waste-to-energy_plant
- WTE International (n.d.) ‘Waste-to-energy technologies overview’. Available at: https://wteinternational.com/news/waste-to-energy-technologies-overview/